Recovering From Spinal Cord Injuries
- Published13 Jan 2015
- Reviewed13 Jan 2015
- Author Mary Bates
- Source BrainFacts/SfN
Every year, between 250,000 and 500,000 people around the world suffer a spinal cord injury, leaving many to face a lifetime of permanent, debilitating symptoms. For years, there was little hope for treatment options. Now scientists are exploring new ways to preserve and rewire nerve tissue and restore function, offering hope for recovery in the future.
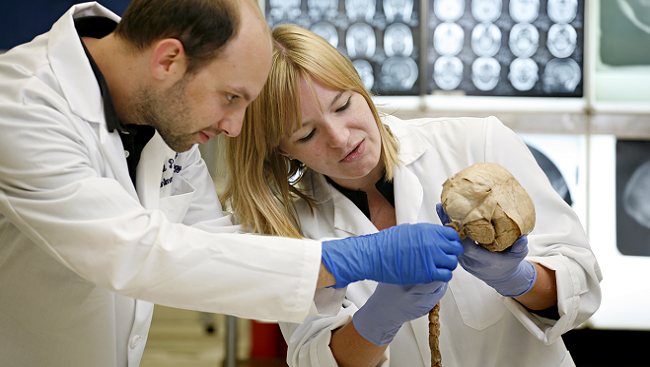
The spinal cord transmits information between the brain and the rest of the body. When it is damaged by trauma or disease, the nerve bundles that carry these important messages can stop working. This can lead to a range of devastating and often permanent symptoms, including the loss of sensation and movement below the point of the injury.
Although there are currently no therapies that can fully restore function after a spinal cord injury, scientists are uncovering new clues about preserving and rewiring nerve fibers and are developing strategies to improve mobility.
Regrowing Nerves
"Repair of the damaged spinal cord is one of the greatest challenges facing the neuroscience community," says James Guest, a neuroscientist who studies spinal cord injury at the University of Miami's Miller School of Medicine. By better understanding how the spinal cord’s response to injury differs from other parts of the body, Guest and other scientists hope to identify new strategies to promote recovery.
One way that spinal cord axons — the nerve bundles that carry signals from the body to the brain and vice-versa — differ from those in a leg or arm is that they do not grow back after injury the way they do elsewhere in the body. In fact, when an injury occurs in peripheral nerves, the nerves outside of the brain and spinal cord, studies show that growth-stimulating proteins flock to the site of injury. In contrast, in the central nervous system, chemicals that actively block axon growth flood the site of injury, preventing nerve regrowth and recovery. Researchers are currently working on a variety of experimental treatments for spinal cord injury that recreate the conditions that promote peripheral nerve recovery.
One such strategy is targeting Schwann cells, a type of glia cell that provides support to peripheral nerve fibers. Schwann cells proliferate after peripheral injury and secrete growth factors to help repair damaged axons. They also produce myelin, the insulation that coats axons and helps speed the transmission of nerve signals. When scientists transplanted Schwann cells into rats at the site of spinal cord injury, they found this treatment helped regrow axons. Guest's laboratory is currently testing whether adding Schwann cells into damaged regions of the spinal cord might also encourage myelin production.
Making Connections
In order to restore movement and sensation, axons must grow from surrounding healthy tissue into the site of injury and then continue on to the brain. Even when researchers are able to stimulate the growth of injured axons, they often find they cannot get the axons to grow beyond the site of injury itself.
To promote this growth, Mark Tuszynski, a neuroscientist at the University of California, San Diego, added nerve cells and growth factors to the injury site and beyond. By leaving behind a trail of bread crumbs to guide axons at points along the spinal cord, for the first time researchers witnessed "axons regenerating into and beyond an injury site," Tuszynski explains.
A Destructive Chain Reaction
Overcoming the primary injury to the spinal cord isn't the only hurdle people with spinal cord injury face. Their bodies must also respond to secondary injury — a destructive cascade of events including inflammation, decreased blood flow, and cell death — that starts immediately after the injury takes place and can continue for weeks. Studies suggest that drugs or interventions that interrupt the cascade of secondary injury, if administered early, could improve nerve cell survival and allow more recovery of movement and sensation to occur.
"One of the major effects of spinal cord injury is to reduce the amount of blood flowing to the injured spinal cord, so if you can improve blood flow, you can save tissue," says Charles Tator, a neurosurgeon at the University of Toronto, who is investigating various strategies to preserve nerves and nerve function in people with spinal cord injuries. His lab has found that increasing blood flow at the site of spinal cord injury leads to less severe injuries.
Other labs are testing drugs that might protect nerve cells from death after spinal cord injury. In rodents, administration of the drug minocycline to the site of a spinal injury preserved axons, prevented tissue loss, and led to significant gains in the ability to move and feel.
Researchers are also testing whether cooling the body temperature of patients — a process known as controlled hypothermia — may improve outcomes for people who have recently suffered a spinal cord injury. Early studies indicate the treatment is safe for people, and those who had therapy displayed less severe injuries one year after their injury than those who did not.
Recruiting Other Networks
Some of the most significant advances in spinal cord injury research have been in the field of neurorehabilitation. Neurorehabilitation uses physical therapy exercises to improve mobility and, when initiated soon after injury, the training can enhance the effectiveness of cellular therapies like growth factors. A new treatment developed by Susan Harkema, a neuroscientist at the University of Louisville, builds on this research and demonstrates the value of neurorehabilitation even years after injury.
Harkema's team of researchers implanted electrodes into the spinal cords of four people with paraplegia who had been paralyzed for more than two years. After surgery, the patients underwent daily treadmill training, in which they were suspended in a harness over a treadmill while a team of researchers supported their legs to help them stand or walk, while the electrodes delivered electrical pulses just below their sites of injury.
The researchers saw results within the first week of treatment. When the electrodes were on, the patients were able to flex their toes, ankles, and knees. Other impairments also improved, such as blood pressure and body temperature regulation, bladder control, and sexual function.
Researchers believe the treatment works because it recruits neural circuits that don’t receive input from the brain, like those involved in the knee-jerk reflex, as well as other neurons that escaped injury. If the injury has spared any neural connections, even if they're not working as well as they were before the injury, the stimulation might "wake up" these connections and help paralyzed muscles move again, Harkema says.
Such results suggest that this type of intervention may prove beneficial to patients even years after injury, and in cases of complete paralysis that were previously thought to be hopeless, she added.
A Promising Future
While there is a wide range of experimental approaches to treating spinal cord injury, they all share a common goal: improving the lives of people with spinal cord injuries.
"What we know about spinal cord injury has dramatically increased in the last 40 years," says Guest. "The rate of acceleration of improvements in treating spinal cord injuries will continue in the next decades, and the outlook for such patients will only get better."
CONTENT PROVIDED BY
BrainFacts/SfN
References
Bradbury EJ, McMahon SB. Spinal cord repair strategies: why do they work? Nature Reviews Neuroscience 7: 644- 653 (2006).
Guest J, Santamaria AJ, Benavides FD. Clinical translation of autologous Schwann cell transplantation for the treatment of spinal cord injury. Current Opinion in Organ Transplantation. 18: 682-289 (2003).
Hall ED, Springer JE. Neuroprotection and acute spinal cord injury: a reappraisal. NeuroRx. 1(1): 80-100 (2004).
Hollis ER II, Tuszynski MH. Neurotrophins: potential therapeutic tools for the treatment of spinal cord injury. Neurotherapeutics. 8: 694-703 (2011).
Lu P, Kadoya K, Tuszynski MH. Axonal growth and connectivity from neural stem cell grafts in models of spinal cord injury. Current Opinion in Neurobiology. 27: 103-109. (2014).
Lu P, Woodruff G, Wang Y, Graham L, Hunt M. Long-distance axonal growth from human induced pluripotent stem cells after spinal cord injury. Neuron. August 7 (2014).
Rossignol S, Schwab M, Schwartz M, Fehlings MG. Spinal cord injury: time to move? Journal of Neuroscience. 27(44): 11782-11792 (2007).
Silva NA, Sousa N, Reis RL, Salgado AJ. From basics to clinical: a comprehensive review on spinal cord injury. Progress in Neurobiology. 114: 25-57 (2014).
Thuret S, Moon LDF, Gage FH. Therapeutic interventions after spinal cord injury. Nature Reviews Neuroscience. 7: 628-643 (2006).
Wells JEA, Hurlbert RJ, Fehlings MG, Yong VW. Neuroprotection by minocycline facilitates significant recovery from spinal cord injury in mice. Brain. 126: 1628-1637 (2003).
Also In Injury
Trending
Popular articles on BrainFacts.org