Restoring Body Functions with Brain-Computer Interfaces
- Reviewed11 Sep 2023
- Author Knvul Sheikh
- Source BrainFacts/SfN
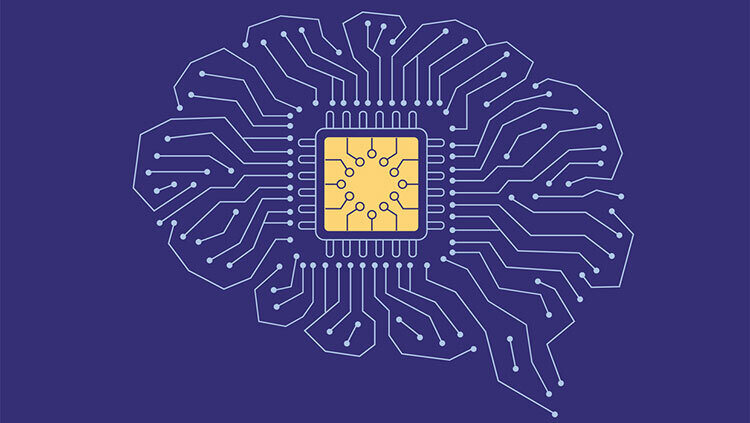
If you know someone with severe neurological damage — amyotrophic lateral sclerosis (ALS), trauma from an accident, a sports or combat injury, or a stroke — you’ve seen firsthand how communicating and performing daily tasks can be a challenge. But during the past few decades, scientists have made significant progress in developing technologies that can restore daily activities and function.
Brain-computer interfaces (BCIs), or brain-machine interfaces, are devices that assist people by translating neuronal information from the brain into commands that control software like applications or hardware like other physical devices. Current brain-machine interfaces can read the activity of millions of neurons through non-invasive means like electroencephalography (EEG) or through more invasive techniques like implanted electrodes. BCIs process these readings and determine which information is important to predict the behavioral intentions of human and animal users. Machine learning techniques then classify and translate this processed information to command a device, giving participants neural control over some of their surroundings — from computer cursors and video games to robotic limbs — without using their muscles.
Despite the sci-fi sizzle, most of the work on electronic brain implants is derived from basic research on how animals and people plan and control various types of movement. As the animals performed repetitive tasks like pressing a lever to receive a reward, researchers found specific electrical patterns associated with the motion. Eventually, computer algorithms were created to translate the patterns that allowed animals to complete a task via a robotic arm or prosthetic device simply by thinking about it.
The clinical applications of brain-machine interfaces quickly became clear. Neuroscientists and surgeons implanted electrode arrays into the brains of people with epilepsy, paralysis, stroke, or Lou Gehrig’s disease (ALS), in hopes of enabling them to communicate and someday, move independently. In early experiments, patients were only able to gain rudimentary control of a computer cursor. But a breakthrough occurred in 2011 when, after months of extensive training, people with quadriplegia learned to control movements of a third (robotic) arm — enabling them to grasp a cup of water or hug a loved one.
Honing this technology could give people the power to control their own paralyzed limbs. Electrode chips implanted in their brains are connected to sleeves or gloves worn over injured limbs. Sending tiny blasts of electricity into the patient’s nerves located under the sleeve or glove can reanimate paralyzed muscles. But brain-machine interfaces won’t become a standard part of clinical medicine until they’re simplified, miniaturized, more affordable, more reliable, and consistently safe and effective. Devices that wirelessly transmit commands from brain implants are a step in that direction.
A parallel line of research has explored applying this technology in the broader field of neuroprostheses. Neuroprosthetic devices include not only devices that receive output commands from a patient's nervous system, but also devices that provide input — as occurs in retinal implants and prosthetic limbs. Prosthetic arms, for example, have remained for the most part low-tech. Although these prosthetics are successful, some brain-guided prostheses have integrated nerves and muscles at several different levels, allowing users to perform more precise and natural movements, and even enabling some to “feel” again.
Still, even the most sophisticated neuroprostheses (such as brain implants) are limited by their number of electrodes and the lifespan of the implanted electrodes. So, a more complex bionic future is still far away. Yet scientists and entrepreneurs are already thinking of new uses for the technology: restoring memory; enhancing cognition; and treating diseases such as depression, Alzheimer’s, and epilepsy. As these technologies have broader uses, people will need to further explore neuroethical issues of privacy, agency, and identity with BCIs.
Adapted from the 8th edition of Brain Facts by Knvul Sheikh.
CONTENT PROVIDED BY
BrainFacts/SfN
References
Armitage, H. (2015). Gene-editing method halts production of brain-destroying proteins. Science. http://www.sciencemag.org/news/2015/10/gene-editing-method-halts-production-brain-destroying-proteins
Belluck, P. (2017). New Electrical Brain Stimulation Technique Shows Promise in Mice. New York Times. https://www.nytimes.com/2017/06/01/health/new-electrical-brain-stimulation-technique-shows-promise-in-mice.html
Boseley, S. (2001). Parkinson’s Miracle Cure Turns into a Catastrophe. The Guardian. https://www.theguardian.com/uk/2001/mar/13/highereducation.education
Chakrabarty, T., Ogrodniczuk, J., & Hadjipavlou, G. (2016). Predictive Neuroimaging Markers of Psychotherapy Response: A Systematic Review. Harvard Review of Psychiatry, 24(6), 396–405. https://doi.org/10.1097/HRP.0000000000000132
Costandi, M. (2015). Gene therapy rescues dying cells in the brains of Alzheimer’s patients. The Guardian. https://www.theguardian.com/science/neurophilosophy/2015/aug/28/gene-therapy-rescues-dying-cells-in-the-brains-of-alzheimers-patients
Deep Brain Stimulation for Movement Disorders. (2017). University of Pittsburgh. http://www.neurosurgery.pitt.edu/centers-excellence/epilepsy-and-movement-disorders-program/deep-brain-stimulation-movement-disorders
Deep Brain Stimulation. (2022). Cleveland Clinic. https://my.clevelandclinic.org/health/treatments/21088-deep-brain-stimulation
Deep Brain Stimulation. (2017). Mayo Clinic. http://www.mayoclinic.org/tests-procedures/deep-brain-stimulation/home/ovc-20156088
Earlier Diagnosis. (2017). Alzheimer’s Association. http://www.alz.org/research/science/earlier_alzheimers_diagnosis.asp
Fisher, E. (2014). Psychiatrists Embrace Deep-Brain Stimulation. Scientific American. https://www.scientificamerican.com/article/psychiatrists-embrace-deep-brain-stimulation/
Gay, M. (2015). Brain New World. The Scientist. http://www.the-scientist.com/?articles.view/articleNo/44079/title/Brain-New-World/
Hardesty, L. (2015). Predicting change in the Alzheimer’s brain. MIT News. Massachusetts Institute of Technology. http://news.mit.edu/2015/predicting-change-alzheimer’s-brain-1006
Kasten, F. H., & Herrmann, C. S. (2017). Transcranial Alternating Current Stimulation (tACS) Enhances Mental Rotation Performance during and after Stimulation. Frontiers in Human Neuroscience, 11, 2. https://doi.org/10.3389/fnhum.2017.00002
Mancuso, L. E., Ilieva, I. P., Hamilton, R. H. and Farah, M. J. (2016). Does Transcranial Direct Current Stimulation Improve Healthy Working Memory?: A Meta-analytic Review. Journal of Cognitive Neuroscience, 28, 8, 1063-1089. https://doi.org/10.1162/jocn_a_00956
Morishita, T., Fayad, S. M., Higuchi, Ma. Et al. (2014). Deep Brain Stimulation for Treatment-resistant Depression: Systematic Review of Clinical Outcomes. Neurotherapeutics, 11, 475–484. https://doi.org/10.1007/s13311-014-0282-1
Nutt, A. E. (2016). The Mind’s Biology. The Washington Post. https://www.washingtonpost.com/sf/national/2016/02/19/brain-hacking-the-minds-biology/
Oremus, W. (2013). Spark of Genius. Slate. http://www.slate.com/articles/technology/superman/2013/04/tdcs_and_rtms_is_brain_stimulation_safe_and_effective.html
Orenstein, D. (2012). People with paralysis control robotic arms using brain-computer interface. Brown University. https://news.brown.edu/articles/2012/05/braingate2
Piore, A. (2015). A Shocking Way to Fix the Brain. MIT Technology Review. https://www.technologyreview.com/s/542176/a-shocking-way-to-fix-the-brain/
Rotsides, J., Mammis, A. (2013). The Use of Deep Brain Stimulation in Tourette’s Syndrome. Medscape. http://www.medscape.com/viewarticle/813944
Schmidt, H. D., Shelton, R. C., & Duman, R. S. (2011). Functional biomarkers of depression: diagnosis, treatment, and pathophysiology. Neuropsychopharmacology, 36(12), 2375–2394. https://doi.org/10.1038/npp.2011.151
Scudellari, M. (2016). Gene Therapy Might Be the Best, and Perhaps Only, Chance at Curing Brain Diseases. Newsweek. http://www.newsweek.com/2016/05/06/gene-therapy-brain-disease-453217.html
She, A. (2016). CRISPR in Neuroscience: How Precision Gene Editing May Unravel How the Brain Works (and Why it Sometimes Doesn’t). SITNBoston. http://sitn.hms.harvard.edu/flash/2016/crispr-in-neuroscience-how-precision-gene-editing-may-unravel-how-the-brain-works-and-why-it-sometimes-doesn’t/
Sheikh, K. (2016). Eavesdropping on the brain. Scienceline. New York University. http://scienceline.org/2016/01/eavesdropping-on-the-brain/
Sheikh, K. (2017). Cell Therapy 2.0: Reprogramming the Brain’s Own Cells for Parkinson’s Treatment. Scientific American. https://www.scientificamerican.com/article/cell-therapy-2-0-reprogramming-the-brain-rsquo-s-own-cells-for-parkinson-rsquo-s-treatment/
Wolters Kluwer Health: Lippincott Williams and Wilkins. (2016). Brain scans could help predict response to psychotherapy for anxiety and depression. ScienceDaily. www.sciencedaily.com/releases/2016/11/161110115340.htm
Zeliadt, N. (2017). Brain Scans May Forecast Autism in Babies. Scientific American. https://www.scientificamerican.com/article/brain-scans-may-forecast-autism-in-babies/