Eliminating Neural Connections
- Reviewed16 Jan 2023
- Author Lindzi Wessel
- Source BrainFacts/SfN
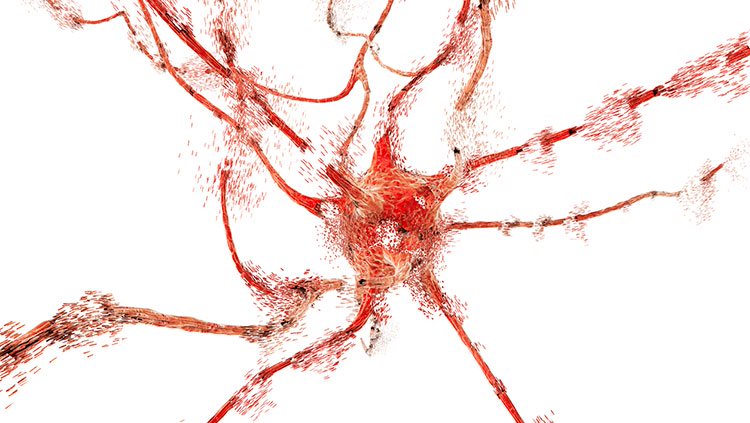
A good gardener prunes trees to help them stay healthy. Similarly, after its initial growth, a neural network is pared back, creating a more efficient system. In fact, only about half the neurons generated during development survive to function in an adult. Entire populations of neurons are removed through apoptosis, a process of programmed cell death initiated in the cells. Apoptosis is activated if a neuron fails to receive enough life-sustaining chemical signals called trophic factors, which are produced in limited quantities by target tissues.
Each type of trophic factor supports the survival of a distinct group of neurons. For example, nerve growth factor is important for the survival of sensory neurons. It has recently become clear that apoptosis is maintained into adulthood but constantly held in check. Based on this, researchers have found that injuries and some neurodegenerative diseases kill neurons not by directly inflicting damage but by activating the cells’ own death programs. This discovery — and its implication that death need not follow insult — have led to new avenues for therapy.
Just as too many brain cells develop early on, these cells initially form an excessive number of connections. In primates, for example, neural projections from the two eyes to the brain initially overlap; then, in some portions of the brain, they sort into separate territories devoted to one eye or the other. Furthermore, connections between neurons in a young primate’s cerebral cortex are more numerous and twice as concentrated as in an adult primate.
The pruning of these excess connections is heavily dependent on the relative activity of each connection. Connections that are active and generating electrical currents survive, while those with relatively little activity are lost. Astrocytes and other glia also play an important role in this process. For example, astrocytes are known to aid the formation of eye-specific connections by engulfing and eliminating unnecessary synapses. Thus, at least to some extent, the circuits of the adult brain are formed by pruning away incorrect connections to leave only the correct ones.
Adapted from the 8th edition of Brain Facts by Lindzi Wessel.
CONTENT PROVIDED BY
BrainFacts/SfN
References
Clarke, L. E., & Barres, B. A. (2013). Emerging roles of astrocytes in neural circuit development. Nature Reviews Neuroscience, 14(5), 311-321. doi:10.1038/nrn3484
Dabrowski, A., Johnson-Venkatesh, E., & Umemori, H. (2017). Synaptic Development. Conns Translational Neuroscience, 89-111. doi:10.1016/b978-0-12-802381-5.00006-3
Ganguly, K., & Poo, M. (2013). Activity-Dependent Neural Plasticity from Bench to Bedside. Neuron, 80(3), 729-741. doi:10.1016/j.neuron.2013.10.028
Homem, C. C., Repic, M., & Knoblich, J. A. (2015). Proliferation control in neural stem and progenitor cells. Nature Reviews Neuroscience, 16(11), 647-659. doi:10.1038/nrn4021
Kolb, B., & Gibb, R. (2014). Searching for the principles of brain plasticity and behavior. Cortex, 58, 251-260. doi:10.1016/j.cortex.2013.11.012
Lenroot, R. K., & Giedd, J. N. (2006). Brain development in children and adolescents: Insights from anatomical magnetic resonance imaging. Neuroscience & Biobehavioral Reviews, 30(6), 718-729. doi:10.1016/j.neubiorev.2006.06.001
Noctor, S., Cunningham, C., & Kriegstein, A. (2013). Radial Migration in the Developing Cerebral Cortex. Cellular Migration and Formation of Neuronal Connections, 299-316. doi:10.1016/b978-0-12-397266-8.00027-2
Ozair, M. Z., Kintner, C., & Brivanlou, A. H. (2012). Neural induction and early patterning in vertebrates. Wiley Interdisciplinary Reviews: Developmental Biology, 2(4), 479-498. doi:10.1002/wdev.90
Perrimon, N., Pitsouli, C., & Shilo, B. (2012). Signaling Mechanisms Controlling Cell Fate and Embryonic Patterning. Cold Spring Harbor Perspectives in Biology,4(8). doi:10.1101/cshperspect.a005975
Plasticity. (2012, April 1). Brainfacts.org. Retrieved from: http://www.brainfacts.org/brain-basics/brain-development/articles/2012/plasticity/
Pollard, T. (2015). New Light on Growth Cone Navigation. Developmental Cell, 35(6), 672-673. doi:10.1016/j.devcel.2015.12.002
Sala, C., & Segal, M. (2014). Dendritic Spines: The Locus of Structural and Functional Plasticity. Physiological Reviews, 94(1), 141-188. doi:10.1152/physrev.00012.2013
Sorg, B. A., Berretta, S., Blacktop, J. M., Fawcett, J. W., Kitagawa, H., Kwok, J. C., & Miquel, M. (2016). Casting a Wide Net: Role of Perineuronal Nets in Neural Plasticity. The Journal of Neuroscience, 36(45), 11459-11468. doi:10.1523/jneurosci.2351-16.2016
Sun, G. J., Zhou, Y., Stadel, R. P., Moss, J., Yong, J. H., Ito, S., Kawasaki, N. K., Phan, A. T., Oh, J. H., Modak, N., et al. (2015). Tangential migration of neuronal precursors of glutamatergic neurons in the adult mammalian brain. Proc. Natl. Acad. Sci., 112, 9484–9489. doi:10.1073/pnas.1508545112
Werker, J. F., & Hensch, T. K. (2015). Critical Periods in Speech Perception: New Directions. Annual Review of Psychology, 66(1), 173-196. doi:10.1146/annurev-psych-010814-015104