Fish Are Swimming in Happy Pills. Here’s Why That’s Bad.
- Published15 Sep 2021
- Author Alexis Wnuk
- Source BrainFacts/SfN
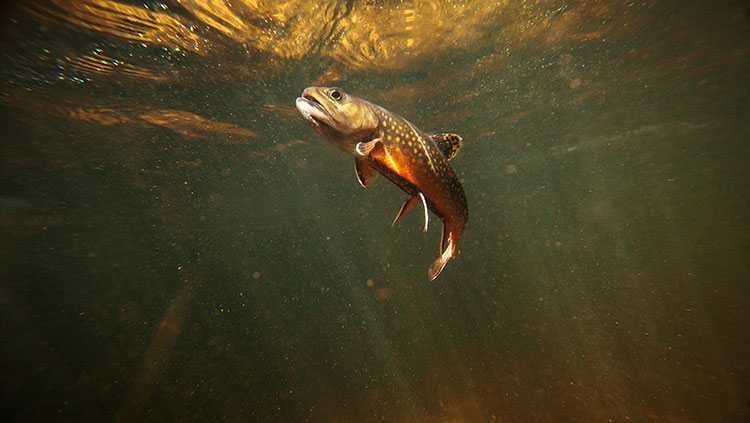
In 1988, Prozac was heralded as a breakthrough. Here was a drug that recalibrated an underperforming serotonin system — at the time, thought to be the root cause of depression — with minimal side effects. Other similar drugs, selective serotonin reuptake inhibitors (SSRIs) soon followed.
Now, more people than ever take antidepressants. The Organization for Economic Cooperation and Development estimates antidepressant consumption doubled between 2000 and 2017 in their 38 member countries. In the U.S., the percentage of people taking antidepressants increased sixfold between 1988 and 2014.
Amid decades of growing use, one subtle side effect has emerged: the drugs are showing up in rivers, lakes, and streams around the world where fish are swimming in them. Even vanishingly small concentrations of these drugs can change fish’s brains and alter their behavior.
“It's been shown for so many different species, and for almost all of the SSRIs, that they have effects at very low concentrations,” says behavioral ecologist Tomas Brodin. “And we find them virtually wherever we take water samples.”
Brodin and a cadre of scientists are sounding the alarm about the growing threat antidepressants and other psychoactive medications pose to wildlife. By changing how fish hunt, evade predators, and mate, these drugs can threaten entire populations and ecosystems. And because we draw drinking water from these very rivers and streams, it may one day affect us as well.
Drugs in the Water
When you swallow an antidepressant pill, only a modest portion of the drug reaches your brain to do its thing. Antidepressants and drugs in general “are not very bioavailable,” says David Constable, Science Director of the American Chemical Society’s Green Chemistry Institute. According to some estimates, less than half of a dose of sertraline, known by the brand name Zoloft, gets into the brain. The rest is flushed out of the body and down the toilet. “Either urine or feces — that’s where most drugs end up,” Constable says.
Drugs also get into the water from improper disposal — flushing pills down the toilet — leaching from landfills, and discharge from pharmaceutical manufacturing facilities.
Sewer pipes shuttle the drug-laced water to wastewater treatment plants, where, in most cases they slip through undetected and unaltered. Conventional wastewater treatment facilities “weren’t designed to destroy pharmaceuticals because we didn’t know about them before,” says Diana Aga, an environmental chemist at the University of Buffalo.
Scientists didn’t know pharmaceutical pollution was a problem because they didn’t have a way to measure the exceedingly small concentrations of drugs in water. “It’s like a drop of ink in an Olympic sized swimming pool,” says Rolf Halden, director of the Center for Environmental Health Engineering at Arizona State University. Only in the last 20 years or so has the technology to quantify such minuscule amounts of water-borne chemicals become widely available. Now, they’re finding drugs everywhere: from urban rivers in the U.S., to a stream in a pristine Australian national park, to coastal waters near Antarctica research stations.
“In the past we have just assumed, ‘oh, we can't see it, so it's probably inconsequential,’” Halden says. “And we now know that that is not true.”
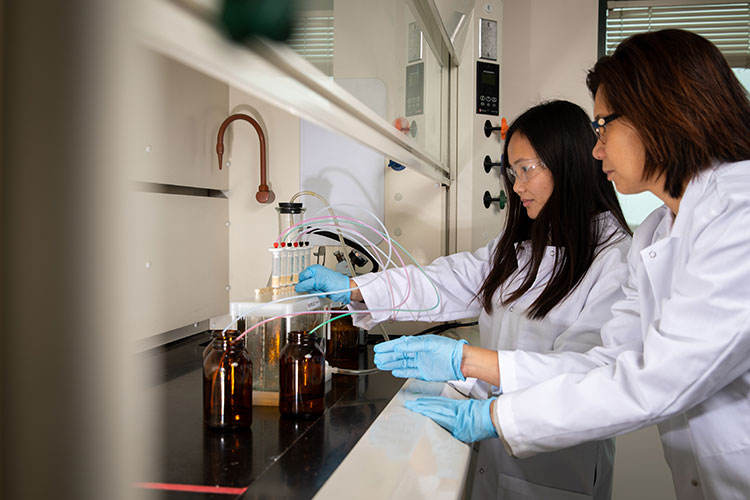
Fish on Chill Phills
In lab experiments, fish on antidepressants act how you might expect: chilled out. Fish in the wild prefer to lurk in the shadows, as swimming out in the open raises their odds of getting nabbed by a predator. But fish on antidepressants swim around more in brightly lit areas of their tanks, unfazed by the potential danger.
And, after a close encounter with a predator, they shrug it off more quickly. In one experiment, behavioral ecologist Bob Wong and his colleagues at Monash University in Australia tested how mosquitofish on fluoxetine (Prozac) responded to a fake attack by a predatory bird. Most fish will remain motionless after potential predatory attacks to avoid further detection, Wong says. To simulate a bird strike, they dropped a small metal rod with a rubber stopper into the tank while cameras recorded the fish’s activity. Fish exposed to tiny doses of fluoxetine spent less time freezing, which might mean they felt less anxious and fearful.
That could be because antidepressants dampen signaling in the brain’s stress response system. In fish, stress kicks off a game of telephone between the hypothalamus, pituitary gland, and inter-renal glands, ultimately leading to a surge of the stress hormone cortisol. (This stress system, called the HPI axis, is analogous to the hypothalamus-pituitary-adrenal axis in humans.) Fish on antidepressants have sluggish HPI axes and less cortisol in their bloodstream, which studies have linked to reduced aggression and fear.
In one of his most recent studies, Wong found guppies on fluoxetine also tended to act more alike. He says that could be really bad. “Variation is key to population viability and survival, particularly in a changing world,” Wong says. “Under normal circumstances maybe a few animals might make bad behavioral decisions and end up getting eaten or suffering lower reproduction.” But if everyone starts making bad decisions, that could jeopardize the entire population.
Fish on antidepressants make lousy hunters, too. In separate studies, fathead minnows and striped bass exposed to fluoxetine took longer to capture prey and ate less overall. By blocking the molecular vacuums that suck up extra serotonin from synapses, SSRIs increase the amount of serotonin available to bind with receptors. Heightened activation of serotonin receptors in the hypothalamus — the brain’s hunger center — dial down appetite and reduce food intake in fish.
The drugs can also disrupt reproduction. In one study, male fathead minnows on fluoxetine turned aggressive to females. If they managed to entice a female to their nest, they often killed her, says Rebecca Klaper, an ecologist at the University of Wisconsin-Milwaukee who led the study. Within a few weeks of continued drug exposure, their behavior shifted. “They kind of mellowed out to the point of really not doing a lot of the same sexual behaviors that they had been doing in the control tanks,” Klaper says.
In Wong’s lab, male mosquitofish on fluoxetine spend more time pursuing females and have higher sperm counts. Still other studies have found antidepressants induce spawning and increase egg-laying and fertility in clams and crayfish.
Altered serotonin signaling in the hypothalamus may once again be to blame. Serotonin stimulates the hypothalamus to release more gonadotropin releasing hormone, or GnRH, which, in turn, prompts the pituitary gland to release reproductive hormones.
Finally, antidepressants may also disrupt learning and memory in some species. In a series of experiments in cuttlefish, researchers in France found the antidepressant venlafaxine — a serotonin and norepinephrine reuptake inhibitor — hampered cell growth in the vertical lobe, a key structure for learning and memory in cephalopods. High doses of the drug impaired cuttlefish’s ability to camouflage itself, which the researchers attributed to impaired processing in the optic lobe.
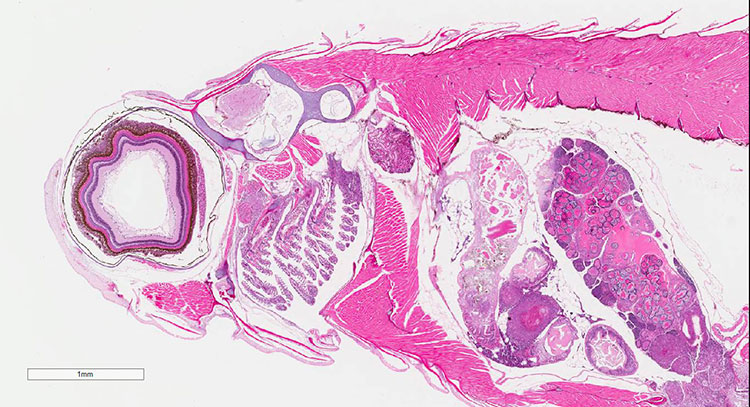
Magnified Effects
It only takes trace amounts of antidepressants to scramble brain signals and change behavior. In Wong’s experiments, water concentrations as low as 18 nanograms per liter — or that drop of ink in an Olympic swimming pool — made fish bolder and more active.
But the scant concentrations don’t tell the whole story. Fish can take up and accumulate way more of these drugs than you’d expect based on the water concentrations. In one of Brodin’s studies on the anti-anxiety drug oxazepam, he found European perch had six times as much of the drug in their muscles compared to the concentration in the stream in which they swam. For invertebrates, the difference is even more dramatic. “Some of the species can have 10- or 20,000-times higher concentrations in their bodies than the water,” says Brodin, a researcher at the Swedish University of Agricultural Sciences. The effect magnifies as you work your way up the food chain. In a 2018 study, he and collaborators estimated that brown trout in an Australian stream consumed as much as 25% of the average human dose of antidepressants every day; platypuses consumed half a dose every day. “There's virtually small pills crawling around on the bottom [of the stream] that the fish and the platypus are feeding on.”
What’s more, fish and insects in the wild don’t encounter a single drug in isolation. “There are hundreds or thousands of these pharmaceuticals in the environment and they interact in all sorts of different ways,” says Michael Bertram, a researcher at the Swedish University of Agricultural Sciences and a colleague of Brodin’s.
Brodin and his team plan to study some of these interactions in their next project. “Not just the mixtures but looking at mixtures that would never be prescribed to humans,” he says. “Because there are a bunch of combinations of drugs … that physicians would never give to an individual because it has terrible effects, but the fish swim in it.”
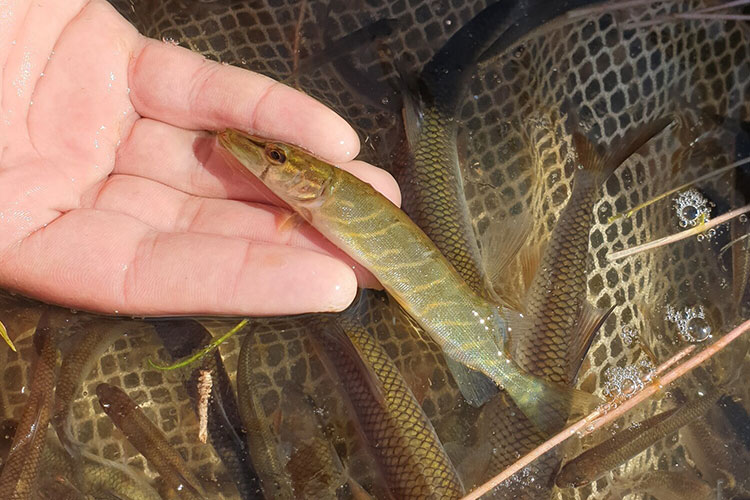
A Solvable Problem
Day in and day out, fish swim in a pharmaceutical soup. The exact drugs and their concentrations can vary from day to day and depend on things like population density, season, and water flow. In her own research, Aga noticed an uptick in the concentration of antidepressants during the COVID-19 pandemic. “It’s a small sample size so I don’t want to over exaggerate it,” she says. “It could also be that, because we’re measuring concentration, it could look higher if the water flow is low.”
“But what I could be very sure about is, in the countries we sample, the U.S. is way higher in concentrations and more types of antidepressants that we detect in water compared to the other countries we sample,” Aga says.
Whatever the cause, we essentially have two options to solve this problem. “We either need to get better with putting fewer problematic chemicals into the wastewater or have really stringent technologies in order to remove those chemicals prior to water reuse,” Halden says.
The former option could involve being more judicious about pharmaceutical use. “These are life-saving chemicals, so we are prepared to have some environmental detriment in order to save lives,” Halden says. “But there also is over-consumption and there's injudicious use of pharmaceuticals, there's abuse of substances.”
Greener drug design could limit the amount of drugs in the environment. Pharmaceuticals are stable by design. No one wants to take a horse pill every 30 minutes, so the pharmaceutical industry responds to that by creating chemistry that doesn’t break down very easily, Halden says. One and done. “The implication is that if something is sturdy enough to survive in our body for 24 hours, then it also … can persist for long periods of time in the environment,” he says.
Developing more biodegradable drugs has been “the holy grail in the drug industry among those of us who are passionate about green chemistry,” says Constable of the American Chemical Society. But it may not be realistic. “There are so many demands on a medicinal chemist, from how you make the drug, to making it bioavailable, to making one that's safe and efficacious,” he says. “To add one more design constraint to say, ‘yeah, we only want this to be stable for a two-year shelf life and the time that it stays in your body and then we want it to fall apart’ — chemically, that's a pretty difficult thing to do.”
That leaves water treatment. Fortunately, the technology to pull pharmaceuticals out of the water already exists. In her research, Aga has identified two strategies that can remove more than 95% of these contaminants during the wastewater treatment process. Ozonation degrades the chemicals to the point where microorganisms can chop them up and destroy them completely, she says. Granular activated carbon can absorb all kinds of contaminants. Just like your Brita filter, though, it needs to be replaced often. Both treatment technologies are expensive and not commonly employed.
Whether it’s an expensive investment in infrastructure or the cost of inaction, one way or another we will pay the price. “We still adhere to this idea that we are separate from the environment and that there's some linear flow where things come and then they leave and they never come back,” Halden says. “But that is not true. There's no Nirvana for wastewater; we are part of the eternal water cycle.”
Indeed, a handful of studies have already documented trace amounts of pharmaceuticals in drinking water, including antidepressants.
If we don’t get smarter about what we’re pumping into the environment, we will suffer the consequences along with other wildlife, Halden says. “We are doomed to live in the chemistry we create.”
CONTENT PROVIDED BY
BrainFacts/SfN
References
Angeles, L. F., Mullen, R. A., Huang, I. J., Wilson, C., Khunjar, W., Sirotkin, H. I., McElroy, A. E., & Aga, D. S. (2019). Assessing pharmaceutical removal and reduction in toxicity provided by advanced wastewater treatment systems. Environmental Science: Water Research & Technology, 6(1), 62–77. https://doi.org/10.1039/C9EW00559E
Arnnok, P., Singh, R. R., Burakham, R., Pérez-Fuentetaja, A., & Aga, D. S. (2017). Selective Uptake and Bioaccumulation of Antidepressants in Fish from Effluent-Impacted Niagara River. Environmental Science & Technology, 51(18), 10652–10662. https://doi.org/10.1021/acs.est.7b02912
Bertram, M. G., Ecker, T. E., Wong, B. B. M., O’Bryan, M. K., Baumgartner, J. B., Martin, J. M., & Saaristo, M. (2018). The antidepressant fluoxetine alters mechanisms of pre- and post-copulatory sexual selection in the eastern mosquitofish (Gambusia holbrooki). Environmental Pollution, 238, 238–247. https://doi.org/10.1016/j.envpol.2018.03.006
Bidel, F., Di Poi, C., Budzinski, H., Pardon, P., Callewaert, W., Arini, A., Basu, N., Dickel, L., Bellanger, C., & Jozet-Alves, C. (2016). The antidepressant venlafaxine may act as a neurodevelopmental toxicant in cuttlefish (Sepia officinalis). Neurotoxicology, 55, 142–153. https://doi.org/10.1016/j.neuro.2016.05.023
Bisesi, J. H., Sweet, L. E., Hurk, P. van den, & Klaine, S. J. (2016). Effects of an antidepressant mixture on the brain serotonin and predation behavior of hybrid striped bass. Environmental Toxicology and Chemistry, 35(4), 938–945. https://doi.org/10.1002/etc.3114
Brodin, T., Fick, J., Jonsson, M., & Klaminder, J. (2013). Dilute Concentrations of a Psychiatric Drug Alter Behavior of Fish from Natural Populations. Science, 339(6121), 814–815. https://doi.org/10.1126/science.1226850
De Pedro, N., Pinillos, M. L., Valenciano, A. I., Alonso-Bedate, M., & Delgado, M. J. (1998). Inhibitory effect of serotonin on feeding behavior in goldfish: Involvement of CRF. Peptides, 19(3), 505–511. https://doi.org/10.1016/s0196-9781(97)00469-5
Emnet, P., Gaw, S., Northcott, G., Storey, B., & Graham, L. (2015). Personal care products and steroid hormones in the Antarctic coastal environment associated with two Antarctic research stations, McMurdo Station and Scott Base. Environmental Research, 136, 331–342. https://doi.org/10.1016/j.envres.2014.10.019
Focazio, M. J., Kolpin, D. W., Barnes, K. K., Furlong, E. T., Meyer, M. T., Zaugg, S. D., Barber, L. B., & Thurman, M. E. (2008). A national reconnaissance for pharmaceuticals and other organic wastewater contaminants in the United States—II) untreated drinking water sources. The Science of the Total Environment, 402(2–3), 201–216. https://doi.org/10.1016/j.scitotenv.2008.02.021
Focazio, M. J., Kolpin, D. W., & Furlong, E. T. (2004). Occurrence of human pharmaceuticals in water resources of the United States: A review. In Pharmaceuticals in the Environment (1st ed., pp. 91–105). Springer. https://pubs.er.usgs.gov/publication/70164321
Ford, A. T., & Fong, P. P. (2016). The effects of antidepressants appear to be rapid and at environmentally relevant concentrations. Environmental Toxicology and Chemistry, 35(4), 794–798. https://doi.org/10.1002/etc.3087
Fursdon, J. B., Martin, J. M., Bertram, M. G., Lehtonen, T. K., & Wong, B. B. M. (2019). The pharmaceutical pollutant fluoxetine alters reproductive behaviour in a fish independent of predation risk. Science of The Total Environment, 650, 642–652. https://doi.org/10.1016/j.scitotenv.2018.09.046
Gaworecki, K. M., & Klaine, S. J. (2008). Behavioral and biochemical responses of hybrid striped bass during and after fluoxetine exposure. Aquatic Toxicology (Amsterdam, Netherlands), 88(4), 207–213. https://doi.org/10.1016/j.aquatox.2008.04.011
Giggs, R. (2019, April 12). Human Drugs Are Polluting the Water—And Animals Are Swimming in It. The Atlantic. https://www.theatlantic.com/magazine/archive/2019/05/pharmaceutical-pollution/586006/
Harvey, M. (2013, March 15). Your tap water is probably laced with antidepressants. Salon. https://www.salon.com/2013/03/14/your_tap_water_is_probably_laced_with_anti_depressants_partner/
Kolpin, D. W., Furlong, E. T., Meyer, M. T., Thurman, E. M., Zaugg, S. D., Barber, L. B., & Buxton, H. T. (2002). Pharmaceuticals, Hormones, and Other Organic Wastewater Contaminants in U.S. Streams, 1999−2000: A National Reconnaissance. Environmental Science & Technology, 36(6), 1202–1211. https://doi.org/10.1021/es011055j
Kreke, N., & Dietrich, D. R. (2008). Physiological Endpoints for Potential SSRI Interactions in Fish. Critical Reviews in Toxicology, 38(3), 215–247. https://doi.org/10.1080/10408440801891057
Lepage, O., Larson, E. T., Mayer, I., & Winberg, S. (2005). Serotonin, but not melatonin, plays a role in shaping dominant–subordinate relationships and aggression in rainbow trout. Hormones and Behavior, 48(2), 233–242. https://doi.org/10.1016/j.yhbeh.2005.02.012
Martin, J. M., Bertram, M. G., Saaristo, M., Fursdon, J. B., Hannington, S. L., Brooks, B. W., Burket, S. R., Mole, R. A., Deal, N. D. S., & Wong, B. B. M. (2019). Antidepressants in Surface Waters: Fluoxetine Influences Mosquitofish Anxiety-Related Behavior at Environmentally Relevant Levels. Environmental Science & Technology, 53(10), 6035–6043. https://doi.org/10.1021/acs.est.9b00944
Martin, J. M., Nagarajan-Radha, V., Tan, H., Bertram, M. G., Brand, J. A., Saaristo, M., Dowling, D. K., & Wong, B. B. M. (2020). Antidepressant exposure causes a nonmonotonic reduction in anxiety-related behaviour in female mosquitofish. Journal of Hazardous Materials Letters, 1, 100004. https://doi.org/10.1016/j.hazl.2020.100004
Martin, J. M., Saaristo, M., Bertram, M. G., Lewis, P. J., Coggan, T. L., Clarke, B. O., & Wong, B. B. M. (2017). The psychoactive pollutant fluoxetine compromises antipredator behaviour in fish. Environmental Pollution, 222, 592–599. https://doi.org/10.1016/j.envpol.2016.10.010
Martin, J. M., Saaristo, M., Tan, H., Bertram, M. G., Nagarajan-Radha, V., Dowling, D. K., & Wong, B. B. M. (2019). Field-realistic antidepressant exposure disrupts group foraging dynamics in mosquitofish. Biology Letters, 15(11), 20190615. https://doi.org/10.1098/rsbl.2019.0615
Painter, M. M., Buerkley, M. A., Julius, M. L., Vajda, A. M., Norris, D. O., Barber, L. B., Furlong, E. T., Schultz, M. M., & Schoenfuss, H. L. (2009). Antidepressants at environmentally relevant concentrations affect predator avoidance behavior of larval fathead minnows (Pimephales promelas). Environmental Toxicology and Chemistry, 28(12), 2677–2684. https://doi.org/10.1897/08-556.1
Pérez-Maceira, J. J., Otero-Rodiño, C., Mancebo, M. J., Soengas, J. L., & Aldegunde, M. (2016). Food intake inhibition in rainbow trout induced by activation of serotonin 5-HT2C receptors is associated with increases in POMC, CART and CRF mRNA abundance in hypothalamus. Journal of Comparative Physiology. B, Biochemical, Systemic, and Environmental Physiology, 186(3), 313–321. https://doi.org/10.1007/s00360-016-0961-9
Pharmaceuticals in the Water Environment. (2010). National Association of Clean Water Agencies.
Polverino, G., Martin, J. M., Bertram, M. G., Soman, V. R., Tan, H., Brand, J. A., Mason, R. T., & Wong, B. B. M. (2021). Psychoactive pollution suppresses individual differences in fish behaviour. Proceedings of the Royal Society B: Biological Sciences, 288(1944), 20202294. https://doi.org/10.1098/rspb.2020.2294
Richmond, E. K., Rosi, E. J., Walters, D. M., Fick, J., Hamilton, S. K., Brodin, T., Sundelin, A., & Grace, M. R. (2018). A diverse suite of pharmaceuticals contaminates stream and riparian food webs. Nature Communications, 9(1), 4491. https://doi.org/10.1038/s41467-018-06822-w
Schultz, M. M., Furlong, E. T., Kolpin, D. W., Werner, S. L., Schoenfuss, H. L., Barber, L. B., Blazer, V. S., Norris, D. O., & Vajda, A. M. (2010). Antidepressant pharmaceuticals in two U.S. effluent-impacted streams: Occurrence and fate in water and sediment, and selective uptake in fish neural tissue. Environmental Science & Technology, 44(6), 1918–1925. https://doi.org/10.1021/es9022706
Tan, H., Polverino, G., Martin, J. M., Bertram, M. G., Wiles, S. C., Palacios, M. M., Bywater, C. L., White, C. R., & Wong, B. B. M. (2020). Chronic exposure to a pervasive pharmaceutical pollutant erodes among-individual phenotypic variation in a fish. Environmental Pollution, 263, 114450. https://doi.org/10.1016/j.envpol.2020.114450
Valenti, T. W., Gould, G. G., Berninger, J. P., Connors, K. A., Keele, N. B., Prosser, K. N., & Brooks, B. W. (2012). Human therapeutic plasma levels of the selective serotonin reuptake inhibitor (SSRI) sertraline decrease serotonin reuptake transporter binding and shelter-seeking behavior in adult male fathead minnows. Environmental Science & Technology, 46(4), 2427–2435. https://doi.org/10.1021/es204164b
Weinberger, J., & Klaper, R. (2014). Environmental concentrations of the selective serotonin reuptake inhibitor fluoxetine impact specific behaviors involved in reproduction, feeding and predator avoidance in the fish Pimephales promelas (fathead minnow). Aquatic Toxicology, 151, 77–83. https://doi.org/10.1016/j.aquatox.2013.10.012
Also In Diet & Lifestyle
Trending
Popular articles on BrainFacts.org