24-Hour Body Clocks: Circadian Rhythms
- Reviewed28 Oct 2022
- Author Diane A. Kelly
- Source BrainFacts/SfN
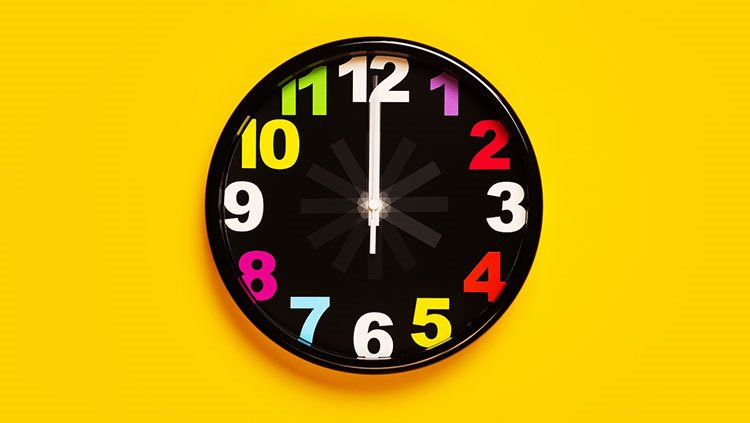
Almost every cell in your body has an internal clock that tells it when to become active, when to rest, and when to divide. These clocks broker changes in many of the body’s physiological systems over a 24-hour, or circadian, period.
For example, the clocks cause faster pulses of peristaltic waves in your gut during the day and make your blood pressure dip at night. But because these clocks are deep inside your body and cannot detect daylight, none of them can tell time on its own. Instead, daily rhythms are coordinated by the suprachiasmatic nucleus (SCN), a tiny group of neurons in the hypothalamus.
Neurons in the SCN act like a metronome for the rest of the body, emitting a steady stream of action potentials during the day and becoming quiet at night. The shift between active and silent states is controlled by cyclic interactions between two sets of proteins encoded by your body’s “clock” genes.
Researchers first identified clock genes in the fruit fly Drosophila melanogaster and studied how they keep time; since then, a nearly identical set of genes has been found in mammals. The SCN also tracks what time it is based on signals it receives from photoreceptors in the retina, which keeps its activity in sync with the Earth’s actual day/night cycle.
That little nudge is very important because, on their own, clock proteins take slightly more than 24 hours to complete a full cycle. Studies of animals deprived of light have discovered that they go to sleep and wake up a bit later each day.
An autonomic neural pathway ties the daily rhythmic activity of the SCN directly to other clocks in the body. Neurons in the SCN stimulate an adjacent region of the brain called the paraventricular nucleus (PVN), which in turn sends signals down a chain of neurons through the spinal cord to the peripheral organs of the body. Signals in part of this neural pathway stimulate orexin neurons to regulate the body’s sleep/wake cycle.
Related pathways also govern the secretion of melatonin, a hormone that influences sleep behaviors. Specifically, electrical activity originating in the SCN enters the PVN’s neural network and sends signals up to the pineal gland, a small pinecone-shaped gland embedded between the cerebral hemispheres. The pineal gland secretes melatonin into the bloodstream at night. Melatonin binds to cells in many tissues, and although it has no direct effect on clock gene expression in the SCN, its systemic effects seem to reduce alertness and increase sleepiness. Light exposure triggers signals that stop melatonin secretion, promoting wakeful behaviors.
Together, these signals keep all the body’s clocks synchronized to the same 24-hour cycle. Coordinated body clocks enable your body’s physiological systems to work together at the right times. When your body prepares to wake from sleep, 1) levels of the stress hormone cortisol peak in the blood, releasing sugars from storage and increasing appetite, and 2) core body temperature begins to drift upwards, raising your body’s metabolic rate. These events, synchronized with others, prepare your body for a new day’s activity.
Desynchronizing the body’s physiological clocks can cause noticeable and sometimes serious health effects. You might have experienced a common example of circadian rhythm disturbance: jet lag. After crossing many time zones in a short period, a person’s patterns of wakefulness and hunger are out of sync with day and night.
Exposure to the local day/night cycle resets the brain and body, but it can take several days to get fully resynchronized. Circadian rhythms can also be disturbed by situations like late-shift jobs or blindness, which decouple normal daylight signals from wake/sleep cycles. Long-term circadian disruptions are associated with health problems, including weight gain, increased rates of insomnia, depression, and cancers.
Adapted from the 8th edition of Brain Facts by Diane A. Kelly.
CONTENT PROVIDED BY
BrainFacts/SfN
References
Aguilera, G. (2011). HPA axis responsiveness to stress: Implications for healthy aging. Experimental Gerontology, 46(2-3), 90–95. https://doi.org/10.1016/j.exger.2010.08.023
Balkaya, M., Prinz, V., Custodis, F., Gertz, K., Kronenberg, G., Kroeber, J., Fink, K., Plehm, R., Gass, P., Laufs, U., & Endres, M. (2011). Stress worsens endothelial function and ischemic stroke via glucocorticoids. Stroke, 42(11), 3258–3264. https://pubmed.ncbi.nlm.nih.gov/21921276/
Carpenter, R., Reddi, B. 2012. Neurophysiology: A Conceptual Approach, Fifth Edition. Hodder Arnold: London.
Costello, R. B., Lentino, C. V., Boyd, C. C., O'Connell, M. L., Crawford, C. C., Sprengel, M. L., & Deuster, P. A. (2014). The effectiveness of melatonin for promoting healthy sleep: a rapid evidence assessment of the literature. Nutrition Journal, 13, 106. https://doi.org/10.1186/1475-2891-13-106
Debono, M., Ghobadi, C., Rostami-Hodjegan, A., Huatan, H., Campbell, M. J., Newell-Price, J., Darzy, K., Merke, D. P., Arlt, W., & Ross, R. J. (2009). Modified-Release Hydrocortisone to Provide Circadian Cortisol Profiles. The Journal of Clinical Endocrinology & Metabolism, 94(5), 1548–1554. https://doi.org/10.1210/jc.2008-2380
Dulac, C. (2010). Brain function and chromatin plasticity. Nature, 465(7299), 728–735. https://doi.org/10.1038/nature09231
Fain, G.L., & O’Dell, T. J. 2014. Molecular and Cellular Physiology of Neurons, Second Edition. Harvard University Press: Cambridge.
Gu, H. F., Tang, C. K., & Yang, Y. Z. (2012). Psychological stress, immune response, and atherosclerosis. Atherosclerosis, 223(1), 69–77. https://doi.org/10.1016/j.atherosclerosis.2012.01.021
Harris, A., & Seckl, J. (2011). Glucocorticoids, prenatal stress and the programming of disease. Hormones and Behavior, 59(3), 279–289. https://doi.org/10.1016/j.yhbeh.2010.06.007
Hasan, K. M., Rahman, M. S., Arif, K. M., & Sobhani, M. E. (2012). Psychological stress and aging: role of glucocorticoids (GCs). Age (Dordrecht, Netherlands), 34(6), 1421–1433. https://doi.org/10.1007/s11357-011-9319-0
Houben, T., Coomans, C. P., & Meijer, J. H. (2014). Regulation of Circadian and Acute Activity Levels by the Murine Suprachiasmatic Nuclei. PLOS ONE, 9(10), e110172. https://doi.org/10.1371/journal.pone.0110172
Klok, M. D., Jakobsdottir, S., Drent, M. L. (2007). The role of leptin and ghrelin in the regulation of food intake and body weight in humans: a review. Obesity Reviews, 8(1), 21–34. https://pubmed.ncbi.nlm.nih.gov/17212793/
Peschel, N., & Helfrich-Förster, C. (2011). Setting the clock – by nature: Circadian rhythm in the fruitfly Drosophila melanogaster. FEBS Letters, 585(10), 1435–1442. https://doi.org/10.1016/j.febslet.2011.02.028
Pfeffer, M., Korf, H. W., & Wicht, H. (2018). Synchronizing effects of melatonin on diurnal and circadian rhythms. General and Comparative Endocrinology, 258, 215–221. https://doi.org/10.1016/j.ygcen.2017.05.013
Purves, D. 2008. Neuroscience, Fourth Edition. Sinauer Associates, Inc.
Reynolds, R. M. (2013). Glucocorticoid excess and the developmental origins of disease: Two decades of testing the hypothesis – 2012 Curt Richter Award Winner. Psychoneuroendocrinology, 38(1), 1–11. https://doi.org/10.1016/j.psyneuen.2012.08.012
Richards, J., & Gumz, M. L. (2012). Advances in understanding the peripheral circadian clocks. The FASEB Journal, 26(9), 3602–3613. https://doi.org/10.1096/fj.12-203554
Skinner, M. K. (2014). Environmental stress and epigenetic transgenerational inheritance. BMC Medicine, 12, 153. https://doi.org/10.1186/s12916-014-0153-y
Sollars, P. J., & Pickard, G. E. (2015). The Neurobiology of Circadian Rhythms. Psychiatric Clinics of North America, 38(4), 645–665. https://doi.org/10.1016/j.psc.2015.07.003
Stojanovich L. (2010). Stress and autoimmunity. Autoimmunity Reviews, 9(5), A271–A276. https://doi.org/10.1016/j.autrev.2009.11.014
Swanson, L. W. 2012. Brain Architecture. 2nd edition. Oxford University Press: Oxford.
Tataroglu, O., & Emery, P. (2014). Studying circadian rhythms in Drosophila melanogaster. Methods, 68(1), 140–150. https://doi.org/10.1016/j.ymeth.2014.01.001
Tawakol, A., Ishai, A., Takx, R. A., Figueroa, A. L., Ali, A., Kaiser, Y., Truong, Q. A., Solomon, C. J., Calcagno, C., Mani, V., Tang, C. Y., Mulder, W. J., Murrough, J. W.,Hoffmann, U., Nahrendorf, M., Shin, L. M., Fayad, Z. A., & Pitman, R. K. (2017). Relation between resting amygdalar activity and cardiovascular events: a longitudinal and cohort study. The Lancet , 389(10071), 834–845. https://doi.org/10.1016/S0140-6736(16)31714-7
What to Read Next
Also In Body Systems
Trending
Popular articles on BrainFacts.org