What Brain Banks Can Teach Us About Alzheimer's
- Published10 Jul 2019
- Author Mirjam Guesgen
- Source BrainFacts/SfN
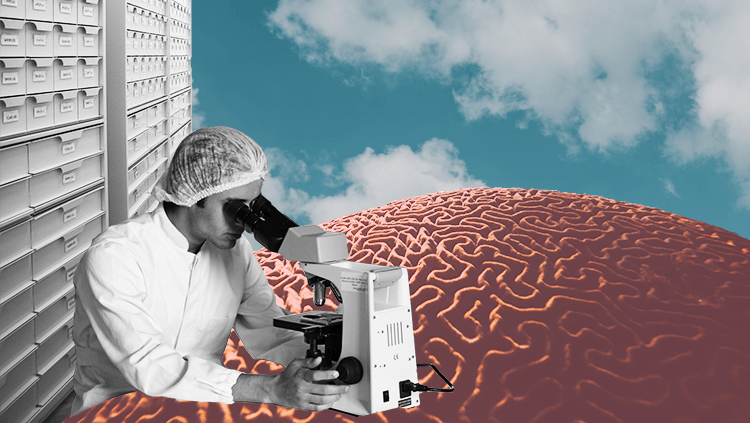
Squeezed between a desk and a stack of textbooks in Jeffrey Joseph’s office are two fairly ordinary looking filing cabinets. They’re loaded with hundreds of slices of human brains. Some are sugar-cube sized chunks preserved in paraffin wax, while others have been cut microns thin and dyed pink, green, and purple before being wedged onto a microscope slide. The pickled specimens make up just a small part of the Calgary Brain Bank.
Joseph, a neuropathologist, heads the bank, a database of more than 100 diseased and healthy brains frozen in time. Stored on meticulously organized shelves and in super-cold freezers, the bank’s samples help scientists study neurodegenerative diseases like Alzheimer’s, with the hopes of diagnosing them earlier and finding potential cures.
Most of the Alzheimer’s research in the last quarter century has hinged on the hypothesis that clumps of deformed proteins eventually kill brain cells and drive the pathology of Alzheimer’s disease. Joseph believes the fixation on these proteins could have put dementia research 30 years behind the rest of neuroscience, “We’re still looking at these proteins and we’ve missed the boat.”
That’s not to say scientists haven’t made tremendous strides in understanding Alzheimer’s disease. They have. But, in many ways we understand Alzheimer’s in animals better than in humans. That’s where brain banks come in. Research at the Calgary Brain Bank and others can bridge the gap between exploratory animal models of Alzheimer’s disease and human clinical trials — and potentially upend decades of conventional wisdom in the process.
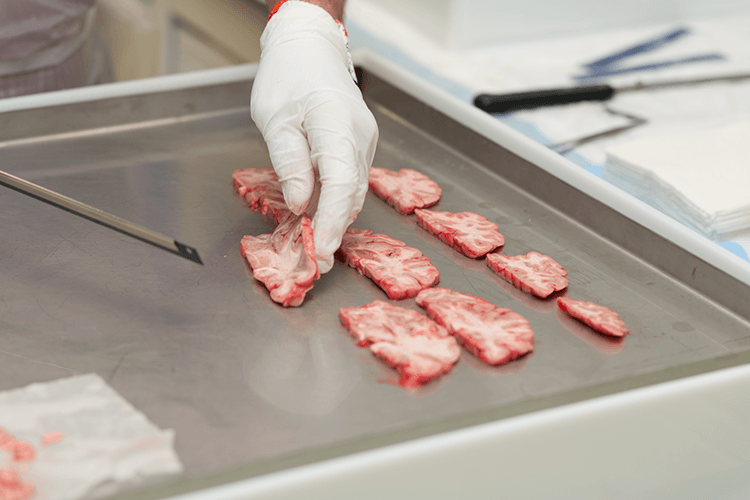
Curing Alzheimer’s in Rodents
Mice are a good starting point for understanding diseases, including Alzheimer’s. Their capacity to breed quickly and the ability to create animals nearly genetically identical to each other make mice a favorite experimental animal for scientists seeking to develop new therapies for diseases including Alzheimer’s.
In fact, scientists have successfully cleared the deformed proteins from the brains of mouse models of Alzheimer’s. But clinical trials of these drugs failed to improve symptoms in people. To date, every therapeutic strategy to attack Alzheimer’s that was successful in rodents has failed in humans.
Alzheimer’s in mice differs from human cases in fundamental ways. For one thing, they’re created by manipulating the mouse’s genes. Most human cases of Alzheimer’s develop without any known genetic mutation. Mice also don’t develop tangles like humans do. “If you’re going to try and [address] the human problem, you’ve got to understand the human issues,” Joseph says.
The Color of Disease
The first step in understanding human Alzheimer’s disease, and diagnosing it early, is to understand the differences between healthy and Alzheimer’s-affected brains. University of Calgary neuroscientist Peter Stys, a regular at the bank, has developed an imaging technique to do just that.
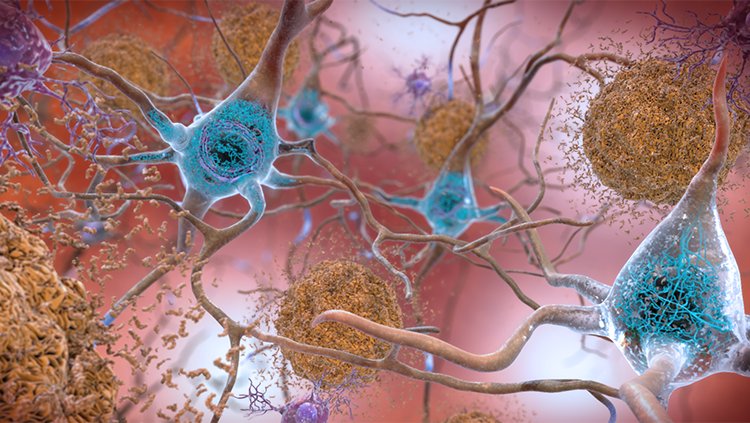
Currently, Alzheimer’s is diagnosed with a series of neuropsychological tests measuring memory, attention, language, and planning. Doctors can use MRI or PET scanning to look for brain shrinkage or hunt for the pathological hallmarks of Alzheimer’s disease — lumps of misfolded proteins called beta-amyloid plaques and tau tangles. The problem is, by the time symptoms like memory loss or trouble planning set in, irreversible brain damage has already been done. Catching the very first signs of Alzheimer’s and diagnosing it earlier means treatment could start sooner, slowing — and one day hopefully halting — the debilitating progression of the disease.
Stys’ imaging technique reveals the chemical structure of plaques and tangles — tau lights up blue whereas amyloid plaques light up green. Minuscule changes in a single protein’s structure, a billionth of a meter small, show up as more subtle color shifts, and the amount of protein clumping is shown by how bright the sample stains.
When Stys used this protein coloring technique on tissue samples from the brain bank, he found that Alzheimer’s-affected tissue stained slightly more yellow-green than healthy tissue, a subtle difference that typical staining techniques would fail to detect. The hue, according to Stys’ doctoral student Anastasiia Stepanchuk, might indicate the presence of smaller, usually invisible, clumps of protein that later bind together into the bigger clumps associated with Alzheimer’s. “These [small deposits] are much harder to detect, unlike big aggregates usually seen on regular histology images,” she explains. And, they might be what’s damaging the brain, Stepanchuk says, so detecting them could be a way to catch Alzheimer’s early.
The same staining technique, with a few minor adjustments, can pick up on these subtle color and brightness changes in white blood cells. Although it’s not clear why white blood cells are altered in Alzheimer’s patients, Stys believes the most plausible explanation is that the cells eat up diseased material, like the small protein clumps, as part of their immune duties. They then carry some of those proteins with them from the brain to the bloodstream where the test can detect them. His hope is that one day everyone over the age of 50 is screened using a blood test like this.
Chemical Sleuthing
While amyloid plaques were discovered in 1906, it wasn’t until the late ʼ80s and early ʼ90s that the amyloid theory of Alzheimer’s took off. That’s when scientists identified genetic mutations linked to inherited, early-onset forms of Alzheimer’s, all of which were involved in the production of beta amyloid. But it isn’t that simple. Healthy people without any Alzheimer’s symptoms can also have plaques and tangles. On the flip side, some people with dementia don’t have these proteins in their brains.
Mirela Ambeskovic came to the Calgary Brain Bank to look for other causes of Alzheimer’s disease. Ambeskovic, a postdoctoral fellow at the University of Lethbridge, and her colleagues Tanzi Hoover, Gerlinde Metz, and Tony Montina, study the chemical fingerprints, called metabolites, left behind when cells perform maintenance and housekeeping duties, like converting food to energy or building proteins. Differences in metabolites between healthy and diseased brains could point to a cellular process or chain of chemical reactions gone awry in disease. For example, Alzheimer’s diminishes the brain’s ability to use glucose as fuel or to assemble proteins from amino acid building blocks. Some of these changes happen before any decline in memory or planning.
Ambeskovic’s project will look at brain areas not typically associated with Alzheimer’s, such as the visual centers. She also plans to investigate whether the degree of metabolic change matches up with records of the person’s symptoms before death. That is, do more drastic differences in metabolites mean the person had more severe memory impairments? Ambeskovic’s work in brain tissue presages the possibility of developing a diagnostic tool that would screen blood, urine, or saliva for specific metabolites.
If we can identify these metabolic changes in blood and urine, we could use them as a “marker for early and faster detection of Alzheimer’s, before neuronal damage and clinical signs are seen,” she says. “Faster detection would mean early treatment implementation and better long-term outcomes for patients.”
Supply and Demand
As researchers like Ambeskovic draw on the reserves of the brain bank to make discoveries, Joseph works to maintain and grow it. It took five years to develop a collection comprised of enough samples for researchers to use. Ensuring the supply is adequate to meet research demands is a constant struggle.
The brain bank acquires samples when families choose to donate the brain of a loved one after an autopsy. “They want to donate because they think it provides some meaning to the death of a loved one,” says Joseph, noting last year 28 families made brain donations — a number that varies widely year to year.
He anticipates that as brain bank researchers make discoveries, more scientists will seek out the bank’s resources.
Joseph points out that there are many avenues in Alzheimer’s research to explore using human tissue. “Dementia work is still in its infancy. I think this is a completely open area. I’m hoping the bank will help.”
CONTENT PROVIDED BY
BrainFacts/SfN
References
Aoyagi, A., Condello, C., Stöhr, J., Yue, W., Rivera, B. M., Lee, J. C., … Prusiner, S. B. (2019). Aβ and tau prion-like activities decline with longevity in the Alzheimer’s disease human brain. Science Translational Medicine, 11(490), eaat8462. doi: 10.1126/scitranslmed.aat8462
Fessel, J. (2018). Amyloid is essential but insufficient for Alzheimer causation: addition of subcellular cofactors is required for dementia. International Journal of Geriatric Psychiatry, 33(1), e14–e21. doi: 10.1002/gps.4730
Jones, M. R., Stys, P. K., Storr, T., & Tsutsui, S. (2016). Development of a diagnostic tool for Alzheimer's disease: Detecting toxic AB species from blood using spectral microscopy. Alzheimer’s & Dementia: The Journal of the Alzheimer’s Association, 12(7), P888–P889. doi: 10.1016/j.jalz.2016.06.1838
Joseph, J. T., Stys, P., Braun, J. E. A., Alvarezveronesi, A., & Smith, E. (2018). The Calgary Brain Bank. Canadian Journal of Neurological Sciences / Journal Canadien Des Sciences Neurologiques, 45(S1), S5–S5. doi: 10.1017/cjn.2018.49
King, A. (2018). The search for better animal models of Alzheimer’s disease. Nature, 559(7715), S13–S15. doi: 10.1038/d41586-018-05722-9
Mehta, D., Jackson, R., Paul, G., Shi, J., & Sabbagh, M. (2017). Why do trials for Alzheimer’s disease drugs keep failing? A discontinued drug perspective for 2010-2015. Expert Opinion on Investigational Drugs, 26(6), 735–739. doi: 10.1080/13543784.2017.1323868
Price, J. L., McKeel, D. W., Jr, Buckles, V. D., Roe, C. M., Xiong, C., Grundman, M., … Morris, J. C. (2009). Neuropathology of nondemented aging: presumptive evidence for preclinical Alzheimer disease. Neurobiology of Aging, 30(7), 1026–1036. doi: 10.1016/j.neurobiolaging.2009.04.002
Roberts, R. O., Aakre, J. A., Kremers, W. K., Vassilaki, M., Knopman, D. S., Mielke, M. M., … Petersen, R. C. (2018). Prevalence and Outcomes of Amyloid Positivity Among Persons Without Dementia in a Longitudinal, Population-Based Setting. JAMA Neurology, 75(8), 970–979. doi: 10.1001/jamaneurol.2018.0629
Rodrigue, K. M., Rieck, J. R., Kennedy, K. M., Devous, M. D., Sr, Diaz-Arrastia, R., & Park, D. C. (2013). Risk factors for β-amyloid deposition in healthy aging: vascular and genetic effects. JAMA Neurology, 70(5), 600–606. doi: 10.1001/jamaneurol.2013.1342
Trushina, E., Dutta, T., Persson, X.-M. T., Mielke, M. M., & Petersen, R. C. (2013). Identification of altered metabolic pathways in plasma and CSF in mild cognitive impairment and Alzheimer’s disease using metabolomics. PloS One, 8(5), e63644–e63644. doi: 10.1371/journal.pone.0063644
Tsutsui, S., Roychoudhury, S., Ota, T., Jones, M. R., Ismail, Z., Cieslak, A., … Stys, P. K. (2016). Detection of Early Alzheimer's disease from blood using novel microspectroscopy. Alzheimer’s & Dementia: The Journal of the Alzheimer’s Association, 12(7), P184–P185. doi: 10.1016/j.jalz.2016.06.321
Watts, J. C., & Prusiner, S. B. (2018). β-Amyloid Prions and the Pathobiology of Alzheimer’s Disease. Cold Spring Harbor Perspectives in Medicine, 8(5), a023507. doi: 10.1101/cshperspect.a023507
Wilkins, J. M., & Trushina, E. (2018). Application of Metabolomics in Alzheimer’s Disease. Frontiers in Neurology, 8, 719–719. doi: 10.3389/fneur.2017.00719