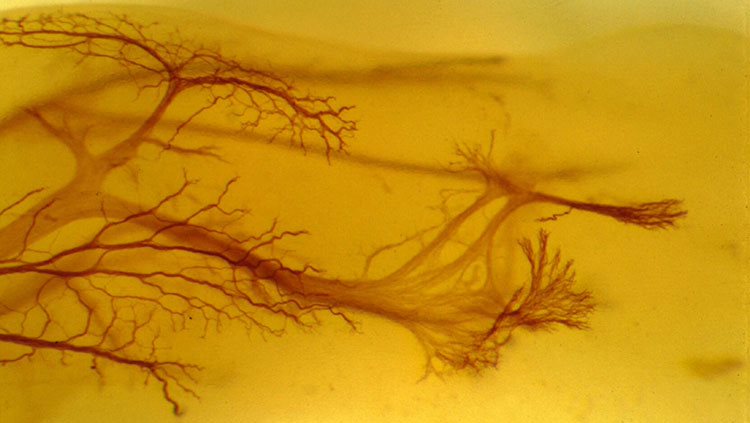
Making new neurons is only the first step of development. Newborn neurons must journey from the inner surface of the embryonic brain, where they formed, to their long-term locations in the brain. This process is called migration, and it begins in humans three to four weeks after a baby is conceived.
At this time, the ectoderm starts to thicken and build up along the midline of the embryo. As the cells continue to divide, a flat neural plate grows, followed by the formation of parallel ridges, somewhat resembling the creases in a paper airplane, that rise along either side of the midline. These ridges extend from the “head end,” where the future brain will form, along the length of the embryo where the future spinal cord will develop.
Within a few days, the ridges fold toward each other and fuse into a hollow neural tube. The head end of the tube thickens into three bulges that form the hindbrain, the midbrain, and the forebrain. Later in the process, at week 7 in humans, the first signs of the eyes and the brain’s hemispheres appear. As new neurons are produced, they move from the neural tube’s ventricular zone, which lies along the inner surface of the tube, toward the border of the marginal zone, or outer surface. After neurons stop dividing, they form an intermediate zone where they gradually accumulate as the brain develops. A variety of guidance cue neurons to migrate to their final destinations.
The most common guidance mechanism, accounting for about 90 percent of migration in humans, is the radial glia, which project radially from the intermediate zone to the cortex. Neurons use these glia as scaffolding, inching along glial projections until they reach their final destinations. This process of radial migration occurs in an “inside-out” manner; that is, the cells that arrive the earliest (the oldest ones) form the deepest layer of the cortex, whereas the late-arriving (youngest) neurons form the outermost layer. Through a different mechanism, other neurons migrate sideways, or tangentially (rather than radially), moving parallel to the brain’s surface and across the radial cortical columns.
Migration is a finely tuned process that can be influenced by many factors. For example, exposure to alcohol, cocaine, or radiation can prevent proper migration, resulting in misplacement of cells, which can lead to intellectual disability or epilepsy. Furthermore, mutations in the genes that regulate migration have been shown to cause rare genetic forms of intellectual disability and epilepsy in humans.
Making Connections
After neurons reach their final locations, they begin making the connections that will determine how particular functions such as vision or hearing can occur. Induction, proliferation, and migration occur internally during fetal development, but the next phases of brain development depend increasingly on external experience. After birth, factors such as watching a mobile spin, listening to a voice, and even proper nutrition influence the connections formed by neurons.
Neurons become interconnected through their short branches called dendrites and long axons — two types of processes that extend from a neuron’s cell body (soma). Axons produce and transmit signals to other neurons, and dendrites receive signals from the axons that contact them.
To reach their targets, axons can span distances many times the size of their cell body, many crossing to the opposite side of the brain. The longest human axons are in the periphery, extending from the lower spinal cord all the way to muscles in the toes. Given the distance from spinal cord to toes of a basketball player — a meter or more — such axons might be nearly a million times longer than their diameter!
A developing axon grows by the extension of its growth cone, an enlargement at the tip of the axon that actively explores the environment to seek out its precise destination. A growth cone is guided to that final destination by molecular cues in its environment. Some of these molecules stud the surfaces of cells, while others are secreted into areas near the growth cone. Receptors on the growth cone enable its responses to these environmental cues. Binding of environmental molecules tells the growth cone whether to move forward, stop, recoil, or change direction. Attractive cues lay a path growth cones follow, while repellent molecules funnel growth cones through precise corridors. Signaling molecules include families of proteins with names such as netrin, semaphorin, and ephrin.
One truly remarkable finding is that most of these proteins are common to many organisms — worms, insects, and mammals including humans. Each family of proteins is smaller in flies or worms than in mice or people, but its functions are very similar. As a result, simpler animals are highly useful experimental models for gaining knowledge that directly applies to humans. For example, netrin was first discovered in a worm, where it was found to guide neurons around the worm’s “nerve ring.” Later, vertebrate netrins were found to guide axons around the mammalian spinal cord. When receptors for netrins were then discovered in worms, this knowledge proved invaluable in finding the corresponding, and related, receptors in humans.
Adapted from the 8th edition of Brain Facts by Lindzi Wessel.
CONTENT PROVIDED BY
BrainFacts/SfN
References
Clarke, L. E., & Barres, B. A. (2013). Emerging roles of astrocytes in neural circuit development. Nature Reviews Neuroscience, 14(5), 311-321. doi:10.1038/nrn3484
Dabrowski, A., Johnson-Venkatesh, E., & Umemori, H. (2017). Synaptic Development. Conns Translational Neuroscience, 89-111. doi:10.1016/b978-0-12-802381-5.00006-3
Ganguly, K., & Poo, M. (2013). Activity-Dependent Neural Plasticity from Bench to Bedside. Neuron, 80(3), 729-741. doi:10.1016/j.neuron.2013.10.028
Homem, C. C., Repic, M., & Knoblich, J. A. (2015). Proliferation control in neural stem and progenitor cells. Nature Reviews Neuroscience, 16(11), 647-659. doi:10.1038/nrn4021
Kolb, B., & Gibb, R. (2014). Searching for the principles of brain plasticity and behavior. Cortex, 58, 251-260. doi:10.1016/j.cortex.2013.11.012
Lenroot, R. K., & Giedd, J. N. (2006). Brain development in children and adolescents: Insights from anatomical magnetic resonance imaging. Neuroscience & Biobehavioral Reviews, 30(6), 718-729. doi:10.1016/j.neubiorev.2006.06.001
Noctor, S., Cunningham, C., & Kriegstein, A. (2013). Radial Migration in the Developing Cerebral Cortex. Cellular Migration and Formation of Neuronal Connections, 299-316. doi:10.1016/b978-0-12-397266-8.00027-2
Ozair, M. Z., Kintner, C., & Brivanlou, A. H. (2012). Neural induction and early patterning in vertebrates. Wiley Interdisciplinary Reviews: Developmental Biology, 2(4), 479-498. doi:10.1002/wdev.90
Perrimon, N., Pitsouli, C., & Shilo, B. (2012). Signaling Mechanisms Controlling Cell Fate and Embryonic Patterning. Cold Spring Harbor Perspectives in Biology,4(8). doi:10.1101/cshperspect.a005975
Plasticity. (2012, April 1). Brainfacts.org. Retrieved from: http://www.brainfacts.org/brain-basics/brain-development/articles/2012/plasticity/
Pollard, T. (2015). New Light on Growth Cone Navigation. Developmental Cell, 35(6), 672-673. doi:10.1016/j.devcel.2015.12.002
Sala, C., & Segal, M. (2014). Dendritic Spines: The Locus of Structural and Functional Plasticity. Physiological Reviews, 94(1), 141-188. doi:10.1152/physrev.00012.2013
Sorg, B. A., Berretta, S., Blacktop, J. M., Fawcett, J. W., Kitagawa, H., Kwok, J. C., & Miquel, M. (2016). Casting a Wide Net: Role of Perineuronal Nets in Neural Plasticity. The Journal of Neuroscience, 36(45), 11459-11468. doi:10.1523/jneurosci.2351-16.2016
Sun, G. J., Zhou, Y., Stadel, R. P., Moss, J., Yong, J. H., Ito, S., Kawasaki, N. K., Phan, A. T., Oh, J. H., Modak, N., et al. (2015). Tangential migration of neuronal precursors of glutamatergic neurons in the adult mammalian brain. Proc. Natl. Acad. Sci., 112, 9484–9489. doi:10.1073/pnas.1508545112
Werker, J. F., & Hensch, T. K. (2015). Critical Periods in Speech Perception: New Directions. Annual Review of Psychology, 66(1), 173-196. doi:10.1146/annurev-psych-010814-015104
What to Read Next
Also In Brain Development
Trending
Popular articles on BrainFacts.org