Mapping Brain Circuits
- Published16 Sep 2009
- Reviewed24 Mar 2014
- Author Debra Speert, PhD
- Source BrainFacts/SfN
Just as Lewis and Clark set out to map the western United States in the early 1800s, neuroscientists are now diligently working to create detailed maps of the major routes — or neural circuits — in the brain.
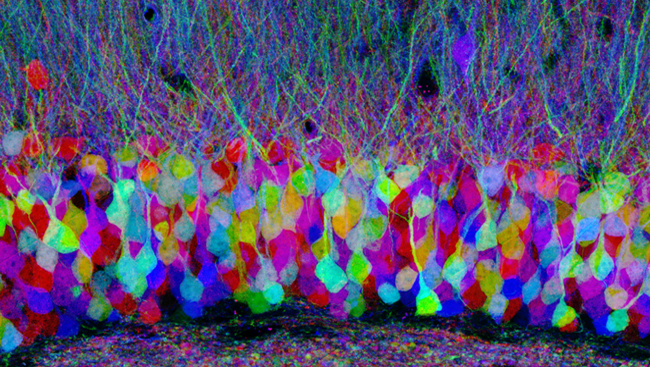
Building a “connectome,” a comprehensive map of the brain’s circuits, is an enormously challenging endeavor, for the brain consists of billions of cells, and each cell contacts thousands of others. But it’s also a very important task, for these circuits will bring scientists one step closer to understanding how the brain functions when healthy and how it fails to function when injured or diseased.
Advanced technologies, along with faster and more data-efficient computers, are making it possible to:
- Trace the connections between individual neurons in animal models.
- Learn more about brain dysfunction in mental health disorders and neurological disease.
Brain cells need each other to get the job done. Every thought, action, and emotion requires the coordinated function of many brain cells working together. But because of technological limitations, researchers are just beginning to learn about the trillions of connections in the brain.
More than 100 years ago, the Italian scientist Camillo Golgi used two chemicals, potassium dichromate and silver nitrate, to stain individual brain cells. This technique enabled scientists to observe entire neurons, including their specialized extensions, axons and dendrites, as they wound their way through brain tissue.
Although Golgi staining ushered in a new era in neuroscience, it was limited to showing only a small number of neurons. In addition, because all cells were stained the same color, their axons and dendrites quickly became indistinguishable from each other under a microscope. Now, new tools are helping to tag and trace cellular connections on a much grander scale.
Seeing cells more clearly
Using a complex genetic engineering technique, scientists have devised a way to label neighboring neurons in different colors, making it easier to differentiate and trace their connections. Called Brainbow for the stunning rainbow-like images it creates, the technique introduces a random mix of red, green, and yellow fluorescent proteins into each neuron. As artists know, combinations of these colors can produce over 100 different hues. Because each neuron gets its own color combination, researchers can better map their connections.
Another technique for mapping neural circuits enlists the power of viruses known to attack and spread through the brain. Recently, scientists modified the rabies virus in a way that enables it to leave behind a green fluorescent protein marker in neurons as it jumps across the synapses (or junctions) between cells.
Other scientists are combining genetic engineering with fiber optics to identify neural circuits. With this technique, flashes of light activate a neuron that has been genetically modified to contain a light-sensing protein. That neuron then stimulates the next neuron in the circuit, so researchers can determine exactly which neurons communicate with each other. This technology may help researchers map and study brain circuits involved in disease. Scientists have already used it to examine disease-related circuitry in studies of Parkinson’s disease in a rodent. Their findings helped explain how a treatment called deep brain stimulation works in people.
The human connectome
Although mapping the precise connections between individual neurons involves research with animals, researchers are using new imaging technologies to examine the human connectome. For example, a noninvasive brain imaging technique that measures water diffusion, called diffusion tensor imaging, can be used to visualize neural circuits connecting localized brain regions. And by comparing diffusion imaging data from healthy and diseased people, researchers are learning how diseases and disorders affect brain circuits.
Yet although we can now look in a comprehensive way at which parts of the human brain are connected to each other, the finer details of how neurons are linked to each other within each part of the brain can be studied — so far, at least — only in experimental animals.
Mapping the connectome is an enormous project, benefiting from the efforts of researchers throughout the neuroscience community. But just as it’s now possible to use Google Earth to track almost any route across the globe, we may one day know how any single neuron communicates with the rest of the brain.
CONTENT PROVIDED BY
BrainFacts/SfN
References
Callaway EM. Transneuronal circuit tracing with neurotropic viruses. Current Opinion in Neurobiology. 18(6):617-623 (2008).
Gradinaru V, Mogri M, Thompson KR, Henderson JM, Deisseroth K. Optical deconstruction of parkinsonian neural circuitry. Science. 324(5925):354-359 (2009).
Livet J, Weissman TA, Kang H, Draft RW, Lu J, et al. Transgenic strategies for combinatorial expression of fluorescent proteins in the nervous system. Nature. 450(7166):56-62 (2007).
Zingg B, Hintiryan H, Gou L, Song MY, Bay M, et al. Neural networks of the mouse neocortex. Cell. 156(5): 1096-1111 (2014).