Smart Moves: Daniel Wolpert, Motor Control, and the Brain
- Published20 Feb 2010
- Reviewed24 Feb 2014
- Source The Kavli Foundation
Think about the word “thinking” and typically it conjures up mulling the meaning of life or plotting your next move in chess. But the brain is also thinking constantly in ways less obvious but equally impressive.
Take that game of chess. Daniel Wolpert, a professor of engineering at the University of Cambridge and a leading researcher on human motor control, admits that an intricate game of chess is an excellent demonstration of the brain at work; however, if you focus less on the game and more on the movement of the pieces, you’ll see something else extraordinary.
While computers are capable of sometimes beating the world’s best (human) chess masters, states Wolpert, “when it comes to dexterity, a five-year-old child could beat any machine being made.”
As Wolpert notes, what gives the brain an edge over artificial computers is its skill at dealing with situations in which outcomes are uncertain and meaningful data is mixed with random noise. At great speed, it compares current sensory data to past experience and weighs probabilities to direct muscles – hundreds of which can be acting at one time — toward a desired action.
In fact, according to Wolpert, this is not only one reason the brain is remarkable; it gets to the root of why we have a brain at all.
A Probabilistic Computer
“We have a brain for one reason and one reason only -- that’s to produce adaptable and complex movements. Movement is the only way we have affecting the world around us… I believe that to understand movement is to understand the whole brain. And therefore it’s important to remember when you are studying memory, cognition, sensory processing, they’re there for a reason, and that reason is action.”Wolpert shared this in his address as the Fred Kavli Distinguished International Scientist Lecture at the Society for Neuroscience meeting: Neuroscience 2009. Later, he and other researchers elaborated on how the brain in its motor-control role is a probabilistic computer, well suited to an uncertain, statistically noisy world. At great speed, Wolpert explained, it compares current sensory data to past experience and weighs probabilities to direct muscles – hundreds of which can be acting at one time -- toward a desired action. And it is able to make decisions long before it gets feedback to see if it has sent the right command. The body “has very long time delays,” Wolpert discussed with the Newsletter. “When you send a command to muscles, it takes a very long time to have an effect.”
So the brain must constantly make educated guesses about what will happen in the next few hundred milliseconds. This is obviously true for difficult physical feats, like returning a well-hit tennis serve, but the process also goes for simple actions, such as picking up a cup. There’s an optimal level of pressure that is just enough to hold the cup so that it doesn’t slip from the fingers, but not so strong that it wastes energy.
“What we’ve shown is that people are extremely accurate at predicting these forces,” says Randy Flanagan, a neuroscientist at Queen’s University in Canada who has worked with Wolpert on studies of motor learning and sensory attenuation (the ability to tune out information caused by one’s own actions). “Humans are not particularly fast, and we’re not particularly strong, and we receive sensations about the world with considerable delay,” Flanagan says. “The way we manage to be successful in the world is through prediction.”
Practice Makes Perfect
The brain learns by doing. The more an action is repeated, the better the brain becomes at anticipating it and sending the right commands. As any piano student knows, repeating the same scales over and over makes performing them smoother and more accurate. The brain’s predictions, hence its commands, are increasingly more on the mark. The question that concerns Wolpert, Flanagan and other students of motor control is how the brain processes information – that is, how it thinks -- to make such movements better and better over time. Their search for answers draws them to the intellectual legacy of an obscure 18th century English clergyman, Thomas Bayes.Bayes is known today for a mathematical formula that calculates the probabilty of an event based on both prior knowledge and new data. Bayes showed that prior probabilities drawn from past observation (such as statistics on disease) have a predictive power that sometimes seems to violate common sense. If we know that 1 out of 1,000 women have breast cancer, for instance, Bayes’ rule tells us that a positive mammogram is still likely to be false even if mammograms are able to detect most cancers. To the conscious mind – especially one untrained in statistics – that outcome may not make sense, but it does seem to make sense to the brain’s motor-control side. Wolpert says the brain appears to think in a Bayesian way as it combines sensory input with prior knowledge of the world to guide our movements.
Wolpert offers the example of how the brain decides where a tennis serve is going to bounce – a prediction that will guide the body’s attempt to return it. This process involves some conscious thought and a great deal of rapid-fire computing in the background. It draws on prior knowledge that includes the memory of past serves, of serves by this opponent in particular and of the sometimes erratic behavior of tennis balls (they don’t always bounce the same way). Sensory data enters the process in the form mostly of visual information – the layout of the court, the detection of irregularities in the surface, the opposing player’s movement and the course of the oncoming tennis ball. This new data can force a revision of prior knowledge as the ball takes an unexpected hop or the opponent has come up with a new spin. But it’s clear from the skill of an experienced player that prior knowledge, as it accumulates, tends to make prediction (and subsequent action) more on target. The brain is constantly calculating Bayesian probabilities and narrowing the scope of uncertainty in doing so.
Tit-for-Tat
A simple experiment in Wolpert’s lab – “one of the dullest,” he calls it – shows how a Bayesian motor-learning process works even in people with no particular physical skill. His subjects, all healthy young adults, sat in front of a screen where a ball appeared at different locations 2000 times. Each time they were asked to point to the spot where the ball had last appeared. Wolpert found that their pointing became more accurate as the experiment proceeded, “suggesting they were able to encode and learn the statistics of where the ball might appear, had knowledge of how variable their visual system was and then combined the two sources of information optimally.”The act of pointing was also a type of decision. It was a very quick and mostly unconscious one, but fundamentally similar to the pattern that humans follow in their conscious minds when they try to choose the best course of action under conditions of limited knowledge. “What’s meant by Bayesian inference, as it applies to decision-making, is something doctors and policy makers do all the time consciously,” says Michael Shadlen, a neurologist and neurobiologist at the Howard Hughes Medical Institute and University of Washington. Shadlen, who collaborated with Wolpert on a 2009 study published in Nature on changes of mind, says he and Wolpert are part of a “sea change” in the way scientists describe the vast range of activities performed by the human brain: “The basic idea is that when you want to talk about things we think of as abstract and cognitive, you have to think about the way the brain controls our bodies.
”The converse is also important, as Wolpert points out: our bodies have plenty of influence over the brain. One’s own physical actions can dramatically affect perception. Just try to tickle yourself — it’s hard to do under normal circumstances — and then see what happens when you tickle someone else. The difference goes back to the predictive behavior of the brain. When the brain decides on a physical action, it anticipates the sensations that will result and discounts their value as information. (It’s more interested in surprises, which force it to revise its prior knowledge and learn something new). “The idea is that, when you try to tickle yourself, the brain makes a prediction and subtracts from the signal.” Wolpert says.
The brain’s power of prediction also helps explain why humans tend to move so much more adeptly than robots. Robots have been held back by their need to wait for feedback from one action before deciding on the next. The brain solves this problem by computing the probable outcomes of future moves before making them. Developing programs to imitate the brain in this way would advance the science of robotics, which Wolpert says is “a bit stuck” at the moment on problems of navigation.
As for benefits on the human side, there are some obvious – if distant – possibilities. Wolpert calls his work “very much blue sky” at this point, but he says it has potential in rehabilitation: “If can we can learn how people do normal learning, we might be able to help people with strokes.” Shadlen, who like Wolpert has a medical degree as well as a PhD, says research on the computational aspect of motor learning could shed light on the circuitry where the computing takes place. That could lead to treatments for diseases of higher brain function -- such as Alzheimer’s, schizophrenia and autism — by enabling Clinicians to locate malfunctioning circuits and replace or repair them. “I’m very hopeful that the principles being discovered in labs like mine,” Shadlen says, “will make it clear what it is that will have to be fixed.”
In the meantime, research on the computational principles of motor control can help us understand everyday occurrences like fights between your kids in the back seat of the family car. A few years ago, Wolpert set out to understand why these battles escalated. Each of his daughters, then age 9 and 12, would always claim that the other one had hit her harder, so they would continue and hit harder each turn. He figured that sensory filtering was at work here, as in tickling: “Whenever you are getting sensations based on your own movements, you will subtract some of that from your own perception. Tit-for-tat actually escalates.” He confirmed the hypothesis with a tapping (not slugging) experiment, finding that the force of the taps increased 40% at each exchange. The same filtering phenomenon explains why people tend not to realize how loudly they’re speaking. These insights may be worth remembering in social situations, childrearing and diplomacy. They also point to a recurring theme in the research by Wolpert, Shadlen, Flanagan and others: The more we know about how the body acts, the more we know about how the brain thinks.
CONTENT PROVIDED BY
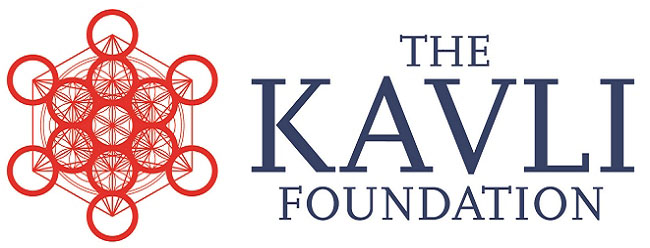
The Kavli Foundation